As the year of quantum (YOQ) amps up, we thought we would take this opportunity reflect on what the quantum community achieved in 2024. This past year has been exceptionally productive and marked by significant advancements. This progress is a result of both the tireless efforts of researchers and the state-of-the-art equipment that supports their work.
Numerous research studies have published results in the past year that bring us closer to practical quantum computing, and the Qblox Cluster and support team provide the tools and solutions needed. In this blog, we share six research papers published in 2024 and discuss how Qblox’s partners have made a tangible impact, helping to bring important quantum discoveries to fruition.
1. Gatemon Qubit on a Germanium Quantum-Well Heterostructure
Researchers from Université Grenoble Alpes and CEA, Grenoble, France, have developed a gatemon qubit using a germanium (Ge) quantum well in a Ge/SiGe heterostructure. Gatemons, a variant of superconducting qubits similar to transmons, feature a gate-tunable semiconducting weak link as the Josephson element. This allows gatemons to offer electrostatic control, potentially overcoming scaling challenges caused by energy dissipation for their flux-controlled conventional counterpart. However, decoherence is a significant hurdle in gatemon development, where the sources for decoherence can be found back in the lossy semiconducting heterostructures and dielectric material.
Elyjah Kiyooka and colleagues infer that their SiGe heterostructure exhibits slightly lower dielectric losses. This advancement likely contributes to the slightly longer relaxation times observed in their gatemon qubits. Their experimental setup featured Qblox's Cluster - including the QCM-RF and QRM-RF modules – to measure their gatemon in pulsed spectroscopy measurements. As this technology lays the groundwork for these advancements, Qblox eagerly anticipates the next steps to push this technology further forward.

Read the paper: https://pubs.acs.org/doi/abs/10.1021/acs.nanolett.4c05539
2. Fast Unconditional Reset and Leakage Reduction in Fixed-Frequency Transmon Qubits
At Chalmers University of Technology, Sweden, researchers developed an innovative protocol for rapidly resetting qubits and minimizing leakage, both of which are essential for efficient quantum error correction. The team focused on fixed-frequency transmon qubits, known for their stability and reliability.
They leverage a tunable coupler to reset the transmon qubit. The coupler unconditionally mediates the transfer of the excited qubit state to the readout resonator, from which it decays into the feedline. The complete transfer process, which includes the unconditional qubit state transfer, decay, and reset of the coupler, happens in just 83 nanoseconds, with a fidelity rate above 99%. The Chalmers experimental setup includes a Qblox control stack, featuring a two-channel signal generator, QCM-RF, for drive pulses, and QRM-RF for readout pulses. Additionally, flux control was managed by a current source S4g and a four-channel QCM.
The outcome of this research not only streamlines the QEC cycle but also significantly enhances algorithmic fidelity. As a result, the developed protocol represents a considerable advancement, facilitating the emergence of more efficient and reliable quantum computing systems. The achievement underscores the potential of fixed-frequency transmon qubits in next-generation quantum architectures compatible with the surface code.
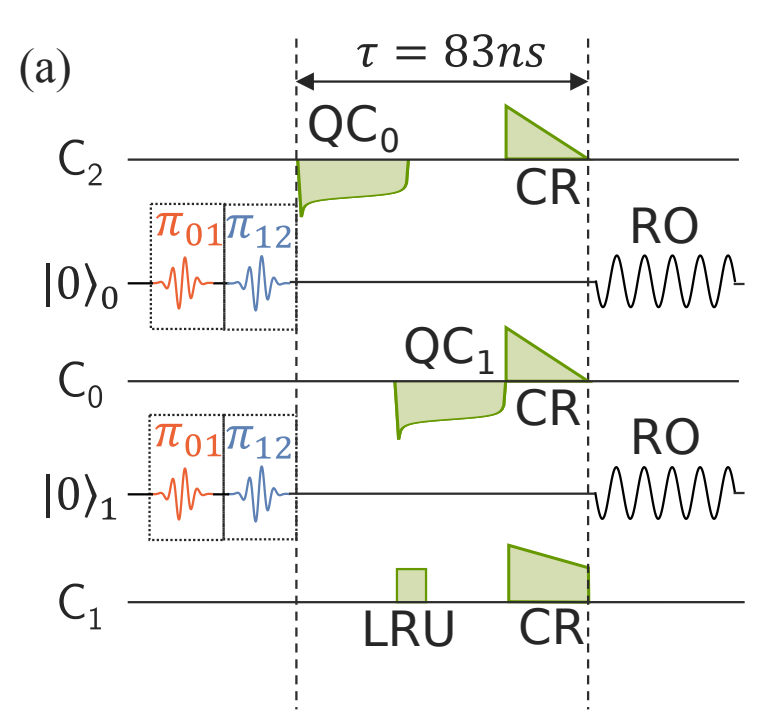
Read the paper: https://arxiv.org/pdf/2409.1674.8
3. High-Fidelity Single-Spin Shuttling in Silicon
Researchers from QuTech, Delft, The Netherlands, have successfully demonstrated high-fidelity shuttling of a single electron spin within a silicon quantum dot array. Silicon-based spin qubits offer advantages in coherence times and integration with existing semiconductor fabrication techniques, making them a promising candidate for large-scale quantum computing.
In their study, the team created a quantum dot array that enabled the transport of an electron spin over an effective distance of 10 micrometers in under 200 nanoseconds, achieving an average fidelity of 99%. This development is important for future quantum processors, where the ability to move qubits efficiently without significant loss of coherence is crucial. The experimental setup utilized Qblox control electronics, including the QCM, QRM, and DC sourcing module for dynamic NCO phase and frequency control, along with low-noise DC voltages, baseband pulses, and RF reflectometry for accurate readout.
This achievement in high-fidelity electron spin shuttling enables the dynamic reconfiguration of qubit connectivity and the integration of quantum information across larger processors. It marks a significant step towards realizing large-scale quantum computers, highlighting the potential of silicon-based spin qubits in creating efficient and scalable quantum computing systems.
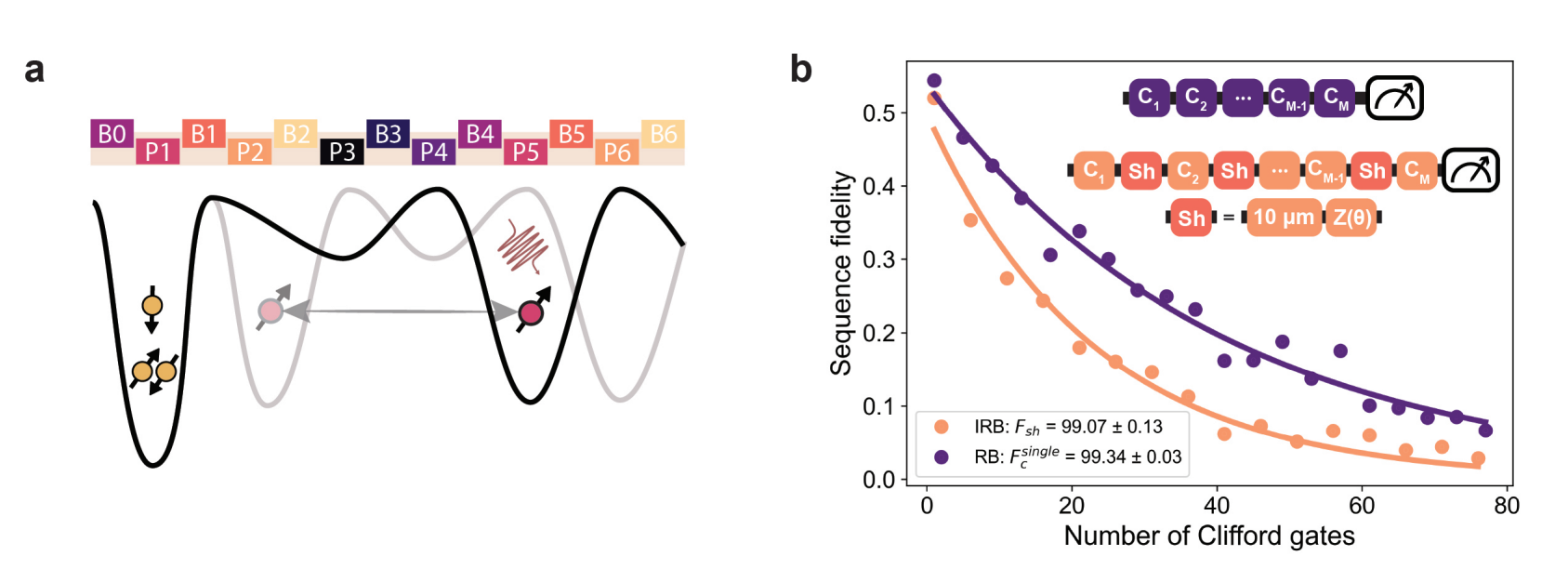
Read the paper: https://arxiv.org/pdf/2406.07267
4. Systematic Study of High EJ/EC Transmon Qudits up to d = 12
At the Department of Physics and Astronomy, University of Rochester, researchers focused on transmons as a platform for qudits, exploiting the higher excited energy levels to encode high-dimensional quantum information. These transmons, designed with a high ratio of Josephson energy (EJ) to charging energy (Ec), give access to manipulating multiple quantum states, opening up new possibilities for quantum information processing. The team recorded observations that indicated up to 12 levels (d = 12), achieving exceptionally low process infidelities between adjacent levels. Even more impressive, they demonstrated high-fidelity control (93.8%) over a 10-dimensional state Hilbert space.
The researchers precisely manipulated and measured these higher energy states using Qblox’s control electronics, including the QRM-RF and QCM-RF modules. What makes this research particularly exciting is the ability to pull off this frequency multiplexed dispersive measurement, with the help of deep neural network analysis, packing more computational power into the same physical footprint. The QRM-RF and QCM-RF support intermediate frequency (IF) multiplexing, thanks to their six independent digital sequencer cores capable of arbitrary waveform generation and data acquisition.
These research achievements in high fidelities within this multi-level approach highlight the potential of qudits as an alternative to qubits. The strong interactions between higher states enable ultra-fast entangling gates, setting the stage for more resource-efficient, scalable quantum systems.
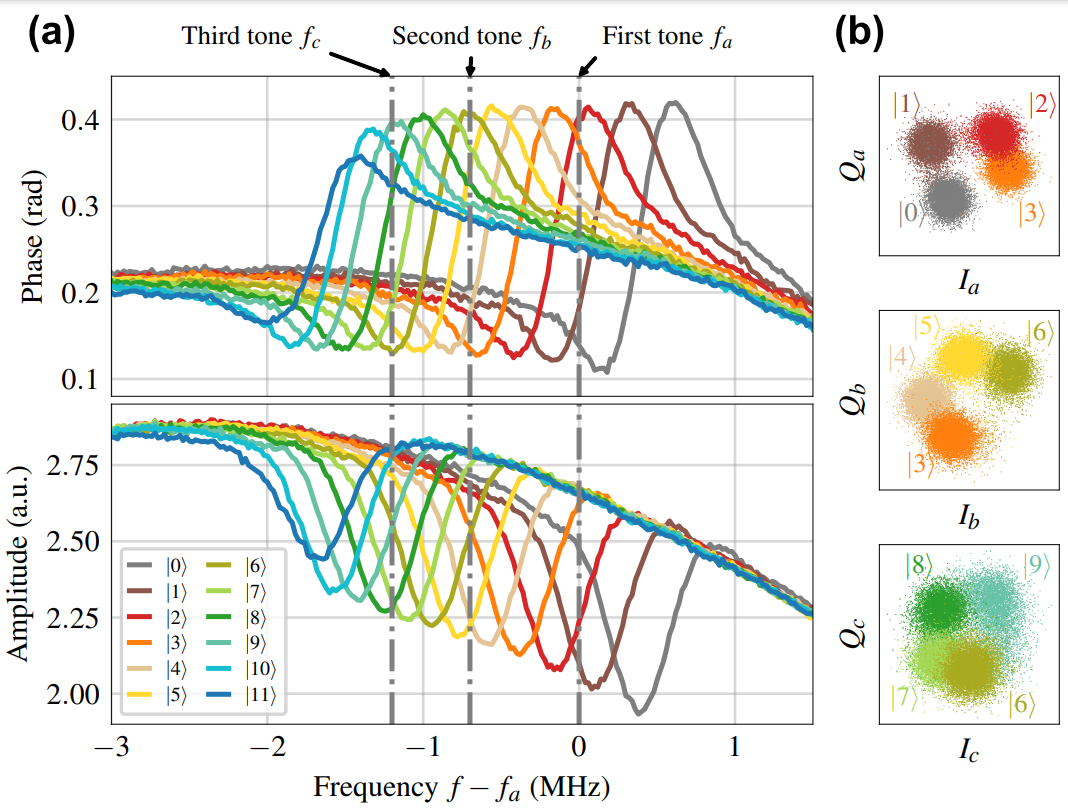
Wang et al., available on arxiv.
Read the paper: https://arxiv.org/pdf/2407.17407
5. Technology and Performance Benchmarks of IQM’s 20-Qubit Quantum Computer
IQM Quantum Computers, a global leader in superconducting quantum computers, has made a pivotal leap in superconducting qubit technology with the introduction of their IQM Crystal 20-qubit quantum processor (aka Garnet). This processor features a square lattice topology and a dedicated tunable coupler solution, enabling high-fidelity two-qubit gate operations.
Powered by the IQM Crystal Quantum Processing Unit (QPU), the system achieves an impressive median two-qubit gate fidelity of 99.5%. A standout achievement in this breakthrough is the successful entanglement of all 20 qubits into a Greenberger–Horne–Zeilinger (GHZ) state.
Integral to the success of this experiment were Qblox’s SPI D5a control electronics, ensuring the necessary ultra-stable and precise flux control for such large-scale entanglement experiments. By providing robust, reliable flux control over the qubits, these electronics played an instrumental role in the success of the development.
This 2024 development marks a significant step toward scalable quantum computing. It not only demonstrates the potential of advanced quantum processors but also brings us closer to practical quantum advantages, paving the way for solving problems that are currently beyond the capabilities of classical computing systems.
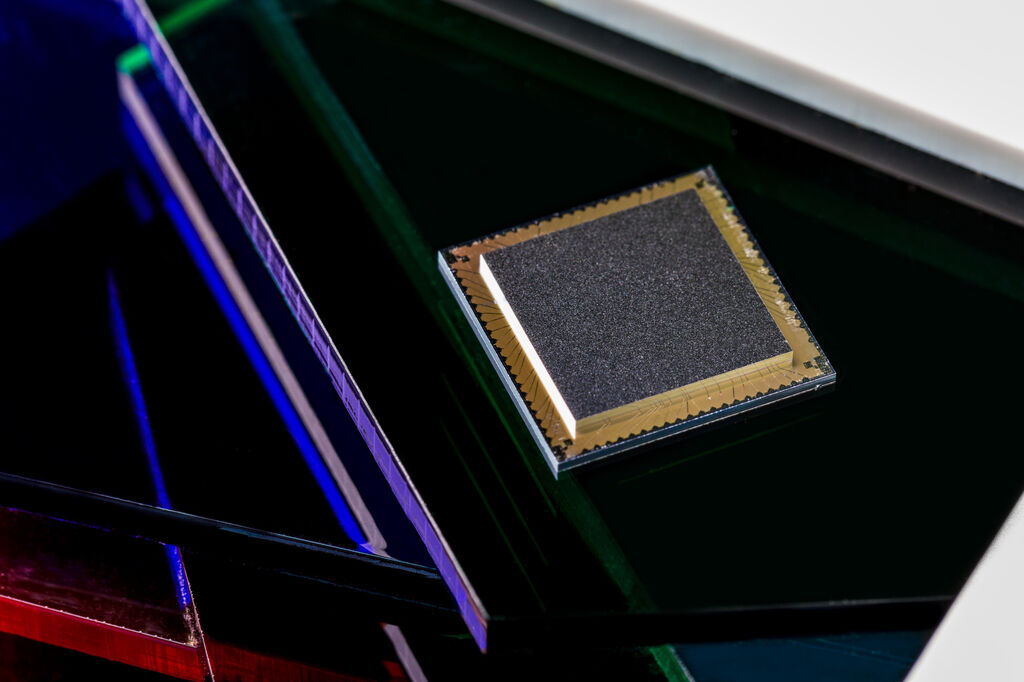
Read the paper: https://arxiv.org/pdf/2408.12433
6. Signal Crosstalk in a Flip-Chip Quantum Processor
Researchers from Chalmers University of Technology in Sweden and VTT Technical Research Centre of Finland have developed a flip-chip superconducting quantum processor featuring exceptionally low signal crosstalk. This design represents an important step toward scalable quantum processors by reducing interference and improving control precision.
Their work demonstrated on-resonant crosstalk levels better than -27 dB (average -37 dB) for capacitively coupled qubit-drive lines and less than 0.13% direct-current flux crosstalk (average 0.05%) for inductively coupled magnetic-flux-drive lines. These results reveal a promising trend: crosstalk decreases with increasing distance, indicating the scalability potential of quantum processors.
The experimental setup utilized Qblox's SPI S4G control electronics, which played a key role in ensuring the robustness and precision of the measurements. The achievement of such low crosstalk levels highlights the strength and flexibility of superconducting circuit architectures, making them a promising foundation for scalable, high-fidelity quantum processors. This advancement is a significant step toward the development of more advanced, practical quantum computing applications, bringing us closer to realizing the full potential of quantum technologies in solving complex real-world problems.
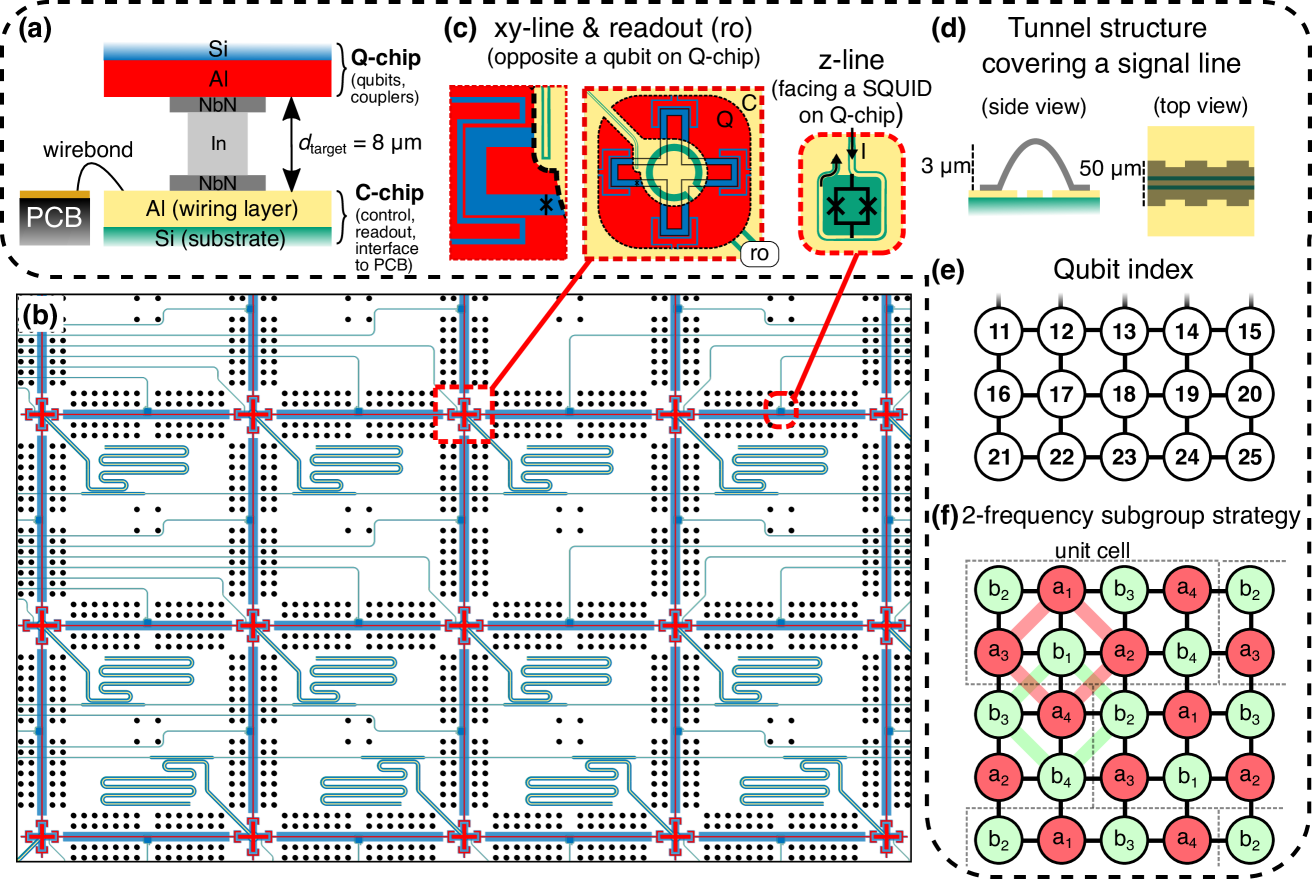
Read the paper: https://journals.aps.org/prxquantum/pdf/10.1103/PRXQuantum.5.030350
Customer support and success are at the heart of what we do at Qblox, and our teams work carefully to ensure that the labs equipped with the Cluster can conduct their research efficiently. Sharing these research papers allows us to highlight significant strides made possible by Qblox's cutting-edge technology. From enabling high-fidelity qubit control in superconducting and silicon-based systems to supporting scalable quantum architectures, Qblox technology facilitated breakthroughs in gatemon qubits, fast qubit reset protocols, high-dimensional qudits, and low-crosstalk quantum processors. With such momentum, the journey ahead promises even greater innovation.
We continue to support groundbreaking discoveries and shape the future of quantum technologies.