Maximizing the performance of quantum devices hinges on the ability to effectively read out and control qubits. At its core, qubit readout involves extracting information about the quantum state, while control focuses on manipulating qubits to perform operations. Take transmons, a type of superconducting qubit, as an example: their readout utilizes frequency multiplexing to address multiple resonators via a single RF line. Control, on the other hand, involves directly coupling the transmon to a microwave drive line that allows for performing single-qubit rotations. Similarly, spin qubits rely on precise coupling to measure phenomena like Pauli spin blockade and to perform control gates via electric dipole spin resonance (EDSR).
A key requirement across these modalities is the necessity for room-temperature control electronics that can generate and measure the signals for qubit readout and control. Although the specific frequencies differ among qubit types, most operate within the range of a few hundred MHz to a few GHz. For instance, superconducting and spin qubits typically use lumped element resonators that require signals in the lower portion of this band, while coplanar waveguide resonators operate in the few-GHz regime.
Clearly, since these frequency requirements for readout and control depend on the precise physical properties of the quantum device and differ from qubit to qubit, and from device to device, it is evident that hardware for readout and control must cover the full frequency range from a few hundred MHz to a few GHz, with channel independence. Before diving into the current solutions for RF signal generation, a question pops up: Have you heard the buzz about Qblox’s QRC module? Is it true that it can cover the full frequency range with independent control of channels, and how?
The art of qubit readout and control
One of the central issues in qubit control is the difficulty of fine-tuning the operating frequency. The ideal frequency is typically determined by device fabrication and global field parameters, making it challenging to control this frequency precisely in situ. Control electronics come into play here, taking on an essential role in qubit control, but also facing high expectations: they need to be highly adaptable and able to handle a wide range of frequencies for each qubit control.
Given that each qubit on the device possesses a unique operating frequency, it is equally important that every frequency in the system can be controlled independently. This includes the ability to adjust any single frequency across its full range while keeping other frequencies constant, or different channels performing their own sweeping pattern completely independently.
Once the ideal operating frequency is identified, frequency stability of the signal becomes the most important metric. Visualized as arrows on a Bloch sphere, qubits rotate around the vertical axis at their specific frequency. To move to the rotating frame of the qubit, it is important that the frequency of the control signal precisely matches the frequency of the qubit. Any drift or noise between them will cause the qubit to dephase and limit the T2 of the qubit. While slow drifts can be corrected with echo sequences, higher frequency phase noise remains uncorrectable and limits qubit performance when either qubit frequency or control signal frequency is noisy. The limitations of control signal frequency on qubit stability have been studied by Biercuk et al. [https://www.nature.com/articles/npjqi201633]. At Qbox, we optimize phase noise and signal-to-noise ratio (SNR) for the best qubit performance. Additionally, we focus on optimizing the Spurious Free Dynamic Range (SFDR) and higher frequency harmonics suppression - both important qubit signal specifications. Any spurious signals present in the output spectrum can directly impact device performance, as these signals can excite neighboring qubits, or perform unwanted qubit rotations in the system. Given these stringent requirements, it becomes imperative to employ advanced RF signal generation techniques.
Current solutions for RF signal generation
The requirements mentioned pose limitations on the way RF signals can be generated. This prompts some key questions: What are the common methods, and are they capable of covering the full frequency range required for readout and control for any qubit modalities?
IQ Mixing
One prevalent method involves using IQ mixers to upconvert frequencies from intermediate frequencies (IF) to the higher frequency required for qubit control. This is achieved by mixing the original signal with a local oscillator (LO) to obtain the RF frequency, following the relation fRF=fint+fLO. The upsides of this method are good phase noise, good Spurious Free Dynamic Range (SFDR), and flexible tunability owing to the fLO adjustable frequency over the entire frequency spectrum. However, the downside of IQ mixing is that all sidebands at frequencies fRF=n · fint + fLO (n = ℕ) are generated due to non-linearities in the mixer. IQ mixer calibration is necessary to remove the first-order unwanted sideband fint + fLO and the LO frequency fLO itself, but cannot address the higher-order sidebands n · fint + fLO , which also appear as a result of the non-linearities. Using a filter to remove these frequencies is impractical as they are close to the desired signal frequency since the intermediate frequency fint is often small.
Double Superheterodyne Setup
An alternative method to eliminate IQ calibration is the double superheterodyne setup. This technique initially upconverts the intermediate frequency fint to a much higher fixed frequency, followed by filtering such that only one of the wanted sidebands remains. The resultant signal is then mixed down to the target RF frequency. Since the two frequencies entering the mixer are now very different in frequency, a fixed bandpass filter is employed to filter out unwanted sidebands and the signals from the two LOs. This method offers the same flexibility as IQ mixing and has an even better signal-to-noise ratio (SNR) spectrum. This comes, however, at the cost of increased phase noise, primarily due to the LO operating at the highest frequency in the signal path. Consequently, the initial upconversion stage results in higher phase noise than the IQ mixing method, making it undesirable for qubit control.
Direct Digital Synthesis (DDS) with RFSoCs
Modern technologies, such as RFSoCs, facilitate the use of direct digital synthesis (DDS). By leveraging higher frequency digital-to-analog converters (DACs) and their higher Nyquist zones, this method can cover a broader frequency range. Furthermore, it can generate signals with low-phase noise due to the absence of analog local oscillators. A challenge here is that, with a fixed sampling rate, there are gaps in the frequency spectrum between the so-called Nyquist zones. While changing the sampling frequency could solve this, it is often impossible for a single channel individually - the channels can no longer be individually controlled independently. The lack of independent control complicates the multiplexing of frequencies with signals from different Nyquist zones with different sampling rates, as signal quality degrades towards the edges of the Nyquist zone. This suboptimal performance at the edges of the Nyquist zones results in low-quality control of the qubits, creating gaps in the frequency spectrum and requiring the end-user to carefully design their quantum experiments to comply with the restrictions imposed by the control electronics.
Can a combined solution cover the full frequency range?
Combining the principles of direct digital synthesis (DDS) and analog mixing technology, Qblox has developed a Quantum Readout and Control (QRC) module that captures the best of both methods. The QRC module employs a combination of DDS and a single mixer scheme, utilizing the higher frequency capabilities of DDS instead of relying on the high-frequency upconversion stage. This approach preserves the calibration-free advantage of the DSH while eliminating the downside of the higher phase noise since the high frequency fLO is no longer needed.
Compared to DDS, the QRC module covers the entire spectrum without any gaps and ensures full channel independence, offering users maximum flexibility. Users only need to specify a center frequency fc, and the hardware will automatically select the proper settings to ensure the full immediate bandwidth is available around this chosen center frequency, for any fc within the module’s output range. This makes the hardware’s limitations invisible to the user, streamlining the process and enhancing usability. Additionally, DDS is used directly whenever possible, with the mixer path employed only to fill the gaps in between. It's up to the user whether they prefer the automated approach or still want to play with DDS and mixing frequencies.
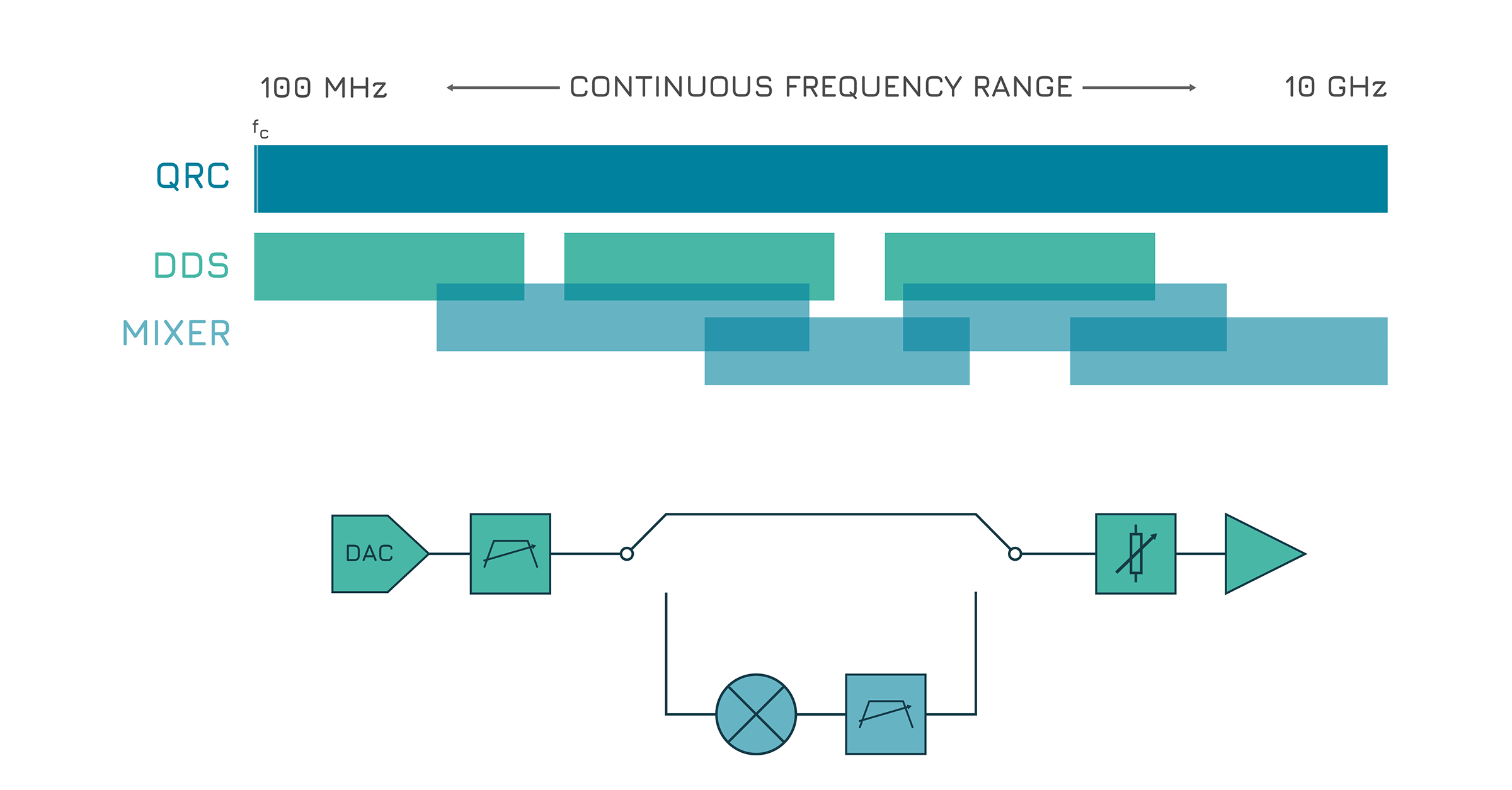
Qblox’s innovative solution: QRC
At Qblox, we are advancing the frontiers with our Quantum Readout and Control (QRC) module, engineered for optimal performance, flexibility, and user-friendly operation. The QRC is a one-of-a-kind highest-density system, thanks to its advanced blend of DDS and parallel analog paths. It supports various qubit types, providing a frequency range from 100 MHz to 10 GHz and up to 80 microwave channels in a single 19” rack. Channel-independent instantaneous bandwidth of >800 MHz is available across the full frequency range, with internal channel-combining doubling bandwidth to >1.6 GHz or frequency multiplex two arbitrarily spaced >800 MHz windows.

The RFSoC-based Q1 real-time sequencer ensures fully deterministic operation, ideal for long pulse sequences and measurement loops. The SYNQ protocol synchronizes all channels under 1 ns with minimal jitter, while the LINQ protocol allows fast, scalable feedback for conditional operations. With gapless playback, measurement cycles avoid unpredictable delays and overheads. The sequencer delivers industry-leading performance with high signal-to-noise and phase stability for improved qubit performance.
Read more about the QRC here.